
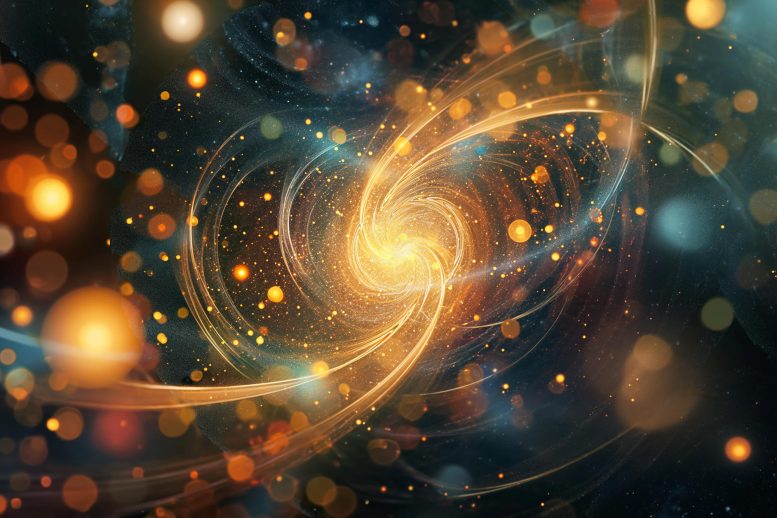
Researchers at Eötvös Lorand University are using advanced particle accelerators to explore the transformation of quark matter in the early universe into ordinary matter. Their innovative techniques and results contribute significantly to our understanding of fundamental physics and the strong interaction. Credit: SciTechDaily.com
Their efforts focused on mapping the “primordial soup” that filled the universe in the first millionth of a second after its creation.
Physicists from Eötvös Loránd University studied the components of the atomic nucleus using the world's three most advanced particle accelerators. Their research aims to explore the “primordial soup” that existed in the universe during the first microseconds after its creation. Interestingly, their findings suggest that the observed particle movement resembles the search for prey of marine predators, climate change patterns, and stock market fluctuations.
In the immediate aftermath of the accident the great explosionThe temperatures were so extreme that neither atomic nuclei nor nucleons, their building blocks, could exist. Hence, in this first case, the universe was filled with a “primordial soup” of quarks and gluons.
As the universe cooled, this medium underwent a “freezing” process, leading to the formation of the particles we know today, such as protons and neutrons. This phenomenon is replicated on a much smaller scale in particle accelerator experiments, where collisions between two nuclei create tiny droplets of quark matter. These droplets eventually transition into ordinary matter through freezing, a transformation known to researchers conducting these experiments.
Differences in quark matter
However, the properties of quark matter differ due to differences in pressure and temperature resulting from collision energy in particle accelerators. This difference requires measurements to “scan” matter at particle accelerators of different energies, such as the Relativistic Heavy Ion Collider (RHIC) in the United States, or the Superproton Collider (SPS) and the Large Hadron Collider (LHC) in Switzerland.
“This aspect is so important that new accelerators are being created all over the world, for example in Germany or Japan, specifically for such experiments. “Perhaps the most important question is how the transition between phases occurs: a critical point may appear on the phase map.” , explains Mati Chanad, Professor of Physics at the Department of Atomic Physics at Eötvös Lorand University (ELTE).
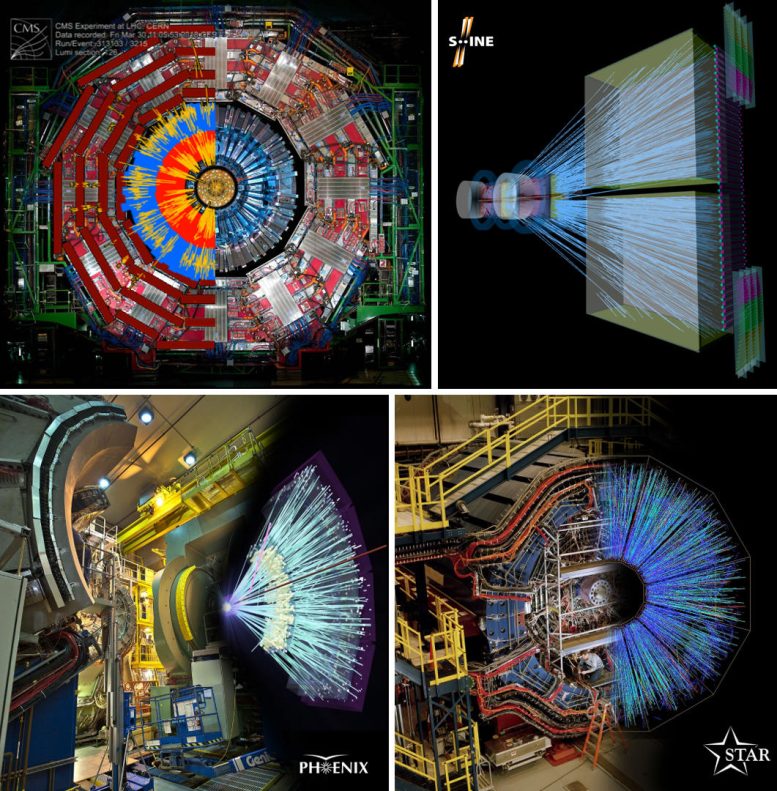
A montage of reconstructed tracks from actual impact events and photographs of the detectors involved, at Brookhaven National Laboratory and at CERN. Source: Montage made by Máté Csanád / Eötvös Loránd University Original images of the montage: STAR és PHENIX: Brookhaven National Laboratory and CMS és NA61: CERN
The long-term goal of the research is to deepen our understanding of the strong interaction that governs interactions in quark matter and in atomic nuclei. Our current level of knowledge in this field can be likened to humanity's understanding of electricity in the Volta, Maxwell, or Faraday eras. While they had an idea of the basic equations, it took a great deal of experimental and theoretical work to develop the technologies that profoundly transformed everyday life, from the light bulb to televisions, telephones, computers and the Internet. Likewise, our understanding of strong interaction is still at an early stage, making research to explore and map it crucial.
Innovations in femoscopy
ELTE researchers have been involved in experiments on each of the accelerators mentioned above, and their work over the past few years has led to a comprehensive picture of the geometry of quark matter. They achieved this by applying femtoscopic techniques. This technique uses correlations that arise from the non-classical, quantum-like wave nature of the particles produced, which ultimately reveal the femtometer structure of the medium, which is the source of the particle emission.
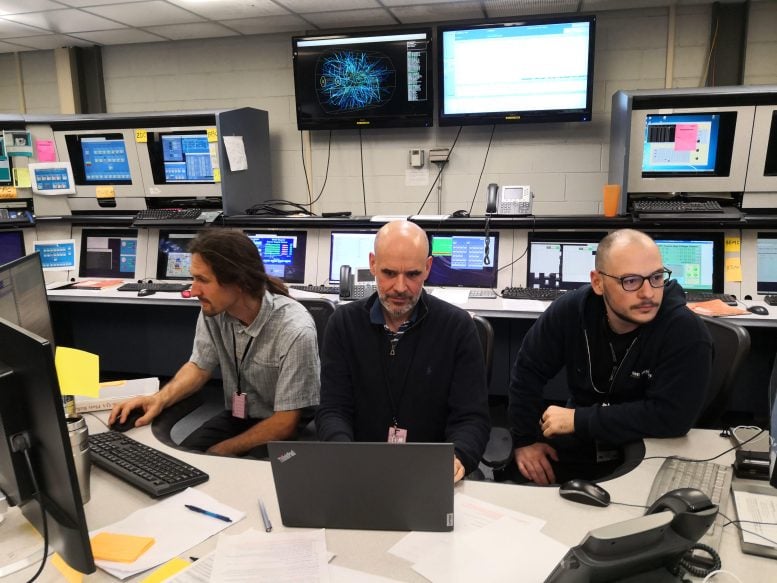
Researchers from Eötvös University work to collect data for the STAR experiment at Brookhaven National Laboratory. Credit: Máté Csanád / Eötvös Loránd University
“In previous decades, femtocopy was performed on the assumption that quark matter follows a normal distribution, i.e. a Gaussian shape found in many places in nature,” explains Marton Nagy, one of the group's lead researchers.
However, Hungarian researchers have turned to Levy's process, also familiar across various scientific disciplines, as a more general framework, which is a good description of the search for prey by marine predators, stock market processes, and even climate change. A distinctive feature of these processes is that at certain moments they undergo very large variations (for example, when a shark searches for food in a new area), and in such cases a Livre distribution can occur instead of a normal (Gaussian) distribution.
Implications and role of ELTE
This research is of great importance for several reasons. First, one of the most studied features of the freezing of quark matter into hadronic matter is the femtoscopic radius (also called the HBT radius, with reference to the well-known Hanbury-Brown and Twyss effect). In astronomy), it is derived from femtoscopic measurements. However, this measure depends on the assumed geometry of the medium. As Daniel Kinsis, a postdoctoral researcher in the group, summarizes, “If the Gaussian assumption is not optimal, the most accurate results from these studies can only be obtained under the Lévy assumption. The Lévy exponent value, which characterizes the Lévy distribution, may They also shed light on the nature of the phase transition, and thus its variation with collision energy provides insight into the different phases of quark matter.
ELTE researchers are actively involved in four experiments: NA61/SHINE at the SPS accelerator, PHENIX and STAR at RHIC, and CMS at the LHC. ELTE's NA61/SHINE group is led by Yoshikazu Nagai, and the CMS group is led by Gabriela Pastor; and the RHIC groups established by Máté Csanád, who also coordinates femtoscopic research at ELTE.
These groups make significant contributions to the success of experiments in various capacities, from reagent development to data acquisition and analysis. They also participate in many theoretical projects and research. “What is unique about our femtoscopy research is that it is being performed in four experiments at three particle accelerators – giving us a broad view into the geometry and possible phases of quark matter,” says Matej Chanad.
Reference: “A New Method for Calculating Bose-Einstein Correlation Functions with Coulomb Final State Interaction” by Marton Nagy, Aleta Borza, Matej Csanad, and Daniel Kinsis, 8 November 2023, European Physical Journal C.
doi: 10.1140/epjc/s10052-023-12161-y
More Stories
Scientists confirm that monkeys do not have time to write Shakespeare: ScienceAlert
SpaceX launches 23 Starlink satellites from Florida (video and photos)
A new 3D map reveals strange, glowing filaments surrounding the supernova