
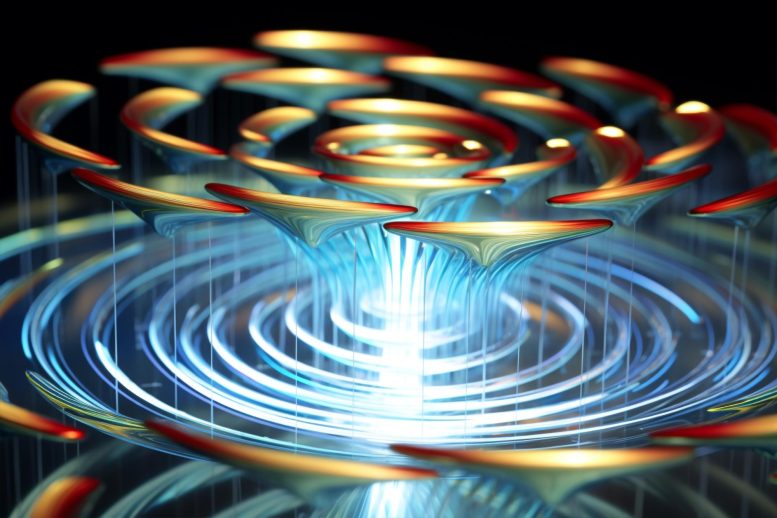
Twistronics is a new field in quantum physics, which involves stacking van der Waals materials to explore new quantum phenomena. Researchers at Purdue University have advanced this field by introducing quantum spin into twisted bilayers of antimagnets, resulting in tunable moiré magnetism. This achievement points to new materials for spin electronics and promises advances in memory devices and spin logic. Credit: SciTechDaily.com
Purdue University researchers spin double bilayers of antiferromagnets to demonstrate tunable moiré magnetism.
Twistronics is not a new dance move, exercise equipment, or music fad. No, it's much cooler than anything like that. It's an exciting new development in quantum physics and materials science where van der Waals materials are stacked on top of each other in layers, like sheets of paper in a stack that can easily twist and turn while remaining flat, and quantum physicists have used these stacks to discover interesting quantum phenomena.
By adding the concept of quantum spin with twisted bilayers of antimagnets, it is possible to have tunable moiré magnetism. This suggests a new class of material platforms for the next step in spinelectronics: spintronics. This new science could lead to promising devices for memory and spin logic, opening the world of physics to a whole new path with spintronics applications.
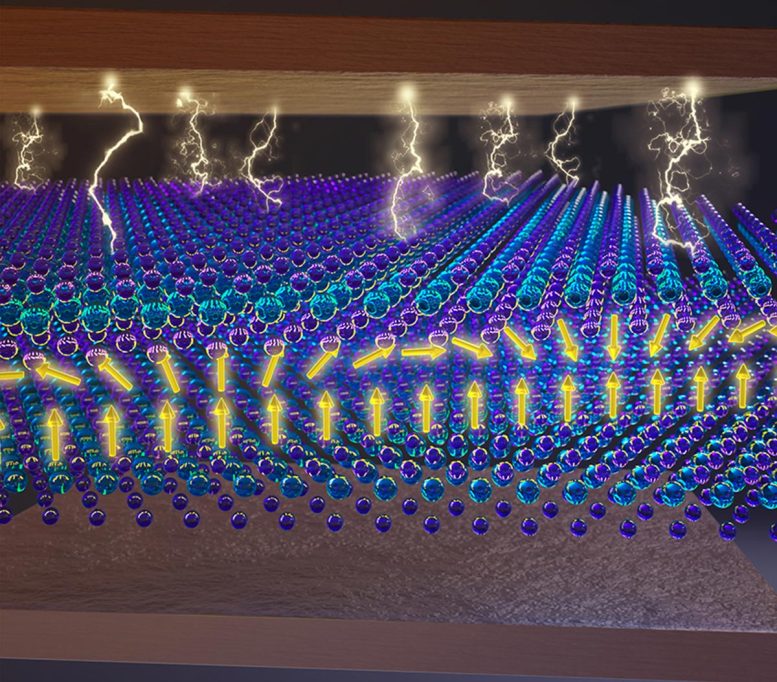
By twisting van der Waals magnets, nonlinear magnetic states with large electrical tunability can emerge. Credit: Ryan Allen, Second Bay Studios
A team of researchers in quantum physics and materials at Purdue University has introduced a torsion technique to control the degree of freedom of rotation using CrI.3, a van der Waals (vdW) material coupled to the antiferromagnetic interlayer, as its mediator. They published their findings, titled “Electrically tunable moiré magnetism in twisted double bilayers of chromium triiodide,” in the journal Nature electronics.
“In this study, we fabricated a twisted double layer of CrI3“That is, a bilayer plus a bilayer with a twisted angle in between,” says Dr. Guangwei Cheng, co-lead author of the publication. “We report moiré magnetism with rich magnetic phases and great tunability by the electrical method.”
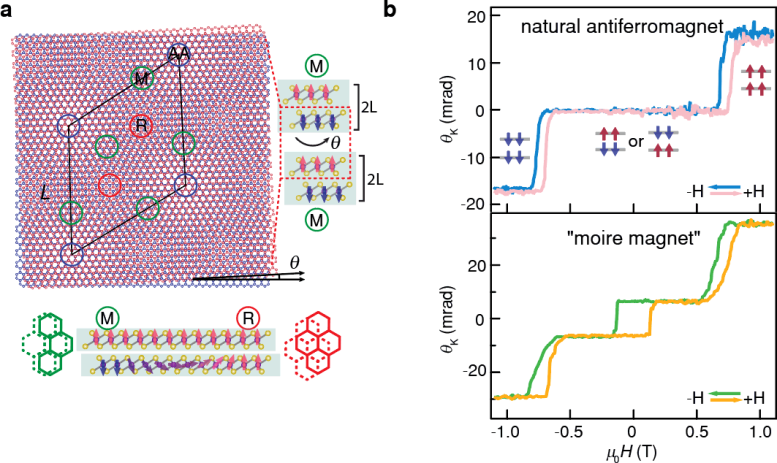
The supermoiré structure of twisted double layer (tDB) CrI3 and its magnetic behaviors investigated by the magneto-optical Kerr effect (MOKE). Section A above shows the schematic diagram of the corrugated superlattice fabricated by twisting the interlayers. Bottom panel: a nonlinear magnetic case can be shown. Section B above shows that the MOKE results demonstrate the coexistence of ferromagnetic (AFM) and ferromagnetic (FM) orders in a tDB CrI3 “moiré magnet” compared to AFM orders in a natural antiferromagnetic CrI3 bilayer. Credit: Illustration by Guanghui Cheng and Yong P. Chen
“We stacked an antiferromagnet and twisted it on itself, and we got a ferromagnet,” Chen says. “This is also a striking example of the recently emerging region of ‘twisted’ magnetism or moiré in 2D twisted materials, where the twist angle between the two layers provides a powerful tuning knob and dramatically changes the material property.”
“For the fabrication of twisted double layer CrI3we tear off one part of the CrI bilayer3“Rotate it and stack it on top of the other part, using what's called the tear and stack technique,” Cheng explains. “By measuring the magneto-optical Kerr effect (MOKE), a sensitive tool for probing magnetic behavior down to a few atomic layers, we observed the coexistence of ferromagnetic and antiferromagnetic orders, the hallmark of moiré magnetism, and further demonstrated the voltage. Auxiliary magnetic switching.Such wave magnetism is a new form of magnetism characterized by spatially varying ferromagnetic and antiferromagnetic phases, alternating periodically according to the moiré superlattice.
To this point, twisttronics has mainly focused on modifying electronic properties, such as the twisted bilayer Graphene. The Purdue team wanted to offer a degree of freedom in rotation and chose to use CrI3, vdW material combined with an antimagnetic layer. The twisting result of stacked antimagnets on themselves is made possible by fabricating samples with different twisting angles. In other words, once fabricated, the torsion angle of each device becomes constant, and MOKE measurements are then performed.
The theoretical calculations for this experiment were performed by Upadhyaya and his team. This provided strong support for the observations made by Chen's team.
“Our theoretical calculations have revealed a phase diagram rich in nonlinear phases of TA-1DW, TA-2DW, TS-2DW, TS-4DW, etc.,” says Upadhyaya.
This research is part of ongoing research by Chen's team. This work follows several recent relevant publications by the team related to the new physics and properties of “2D magnets”, such as “Emergence of electric field tunable interfacial ferromagnetism in 2D magnetic heterostructures“, which was recently published in Nature Communications. This research avenue has exciting potential in the field of spintronics and spintronics.
“The identified corrugated magnets point to a new class of material platforms for spintronics and magnetic electronics,” Chen says. “The observed voltage-assisted magnetic switching and electromagnetic effect may lead to promising memory and spin logic devices. As a new degree of freedom, this twist can be applied to a wide range of homo/hetero bilayers for vdW magnets, opening the opportunity to pursue new physics as well as spintronics applications.”
Reference: “Electrically tunable moiré magnetism in twisted double bilayers of chromium triiodide” by Guanghui Cheng, Muhammad Mushfiqur Rahman, Andres Llacsahuanga Allcca, Avinash Rustagi, Xingtao Liu, Lina Liu, Lei Fu, Yanglin Zhu, Zhiqiang Mao, Kenji Watanabe, Takashi Taniguchi. , Prami Upadhyaya and Yong Pei Chen, June 19, 2023, Nature electronics.
doi: 10.1038/s41928-023-00978-0
The team, mostly from Purdue, includes two equal contributing lead authors: Dr. Guangwei Cheng and Muhammad Mushfiqur Rahman. Cheng was a postdoctoral researcher in Dr. Yong-Pei Chen's group at Purdue University, and is now an assistant professor at the Advanced Institute for Materials Research (AIMR, where Chen is also a principal investigator) at Tohoku University. Muhammad Mushfiqur Rahman is a PhD student in Dr. Prami Upadhyaya's group. Chen and Upadhyaya are corresponding authors of this publication and are professors at Purdue University. Chen is the Carl Lark Horowitz Professor of Physics and Astronomy, professor of electrical and computer engineering, and director of the Purdue Institute for Quantum Science and Engineering. Upadhyaya is an assistant professor of electrical and computer engineering. Other Purdue team members include Andres Laxahuanga Alka (PhD student), Dr. Lina Liu (postdoc), Dr. Li Fu (postdoc) from Chen's group, Dr. Avinash Rustagi (postdoc) from Upadhyaya's group and Dr. Xingtao Leo. (former research assistant at the Burke Center for Nanotechnology).
This work is supported in part by the US Department of Energy (DOE) Office of Science through the Quantum Science Center (QSC, National Quantum Information Science Research Center) and the DoD Multidisciplinary University Research Initiatives (MURI) Program (FA9550-) 20-1 -0322). Cheng and Chen also received partial support from WPI-AIMR, JSPS KAKENHI Basic Science A (18H03858), New Science (18H04473 and 20H04623), and the FRiD Program of Tohoku University in the early stages of the research.
Upadhyaya also acknowledges support from the National Science Foundation (NSF) (ECCS-1810494). bulk cree3 Crystals are provided by the Zhiqiang Mao group of Pennsylvania State University with support from the US Department of Energy (DE-SC0019068). Bulk hBN crystals are provided by Kenji Watanabe and Takashi Taniguchi from the National Institute of Materials Science, Japan, with support from JSPS KAKENHI (grant numbers 20H00354, 21H05233, and 23H02052) and the World Premier Center for International Research Initiative (WPI), MEXT, Japan.
More Stories
Scientists confirm that monkeys do not have time to write Shakespeare: ScienceAlert
SpaceX launches 23 Starlink satellites from Florida (video and photos)
A new 3D map reveals strange, glowing filaments surrounding the supernova